
The resistive heater comprises the graphene nanoribbon and two attached aluminum leads. The thermometers are superconductor–graphene–superconductor (SGS) Josephson junctions that reveal local temperature through the temperature dependence of the switching current, I sw( T) 38. In order to perform thermoelectric measurements, our device additionally contains two thermometers and a resistive heater, fabricated from a graphene monolayer. Two side gate electrodes allow us to tune the resonance levels of the dots independently. 1, consists of an Al superconducting injector in contact with two graphene quantum dots. Several material platforms have been employed in the experiments 30– 37, and particularly promising results have been obtained in carbon nanotube, graphene, and nanowire settings, where the splitting efficiencies approaching 90% have been observed.
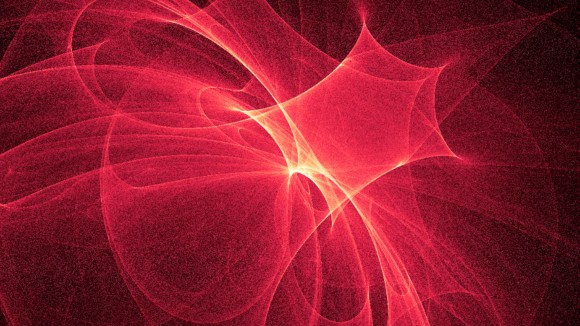
Now, we turn to experimental realization of CPS and EC. The non-local thermoelectric currents in the dots can, in turn, be expressed in terms of the EC and CPS contributions, Δ I L nl = ( Δ I EC + Δ I CPS ) / 2, Δ I R nl = ( − Δ I EC + Δ I CPS ) / 2, where
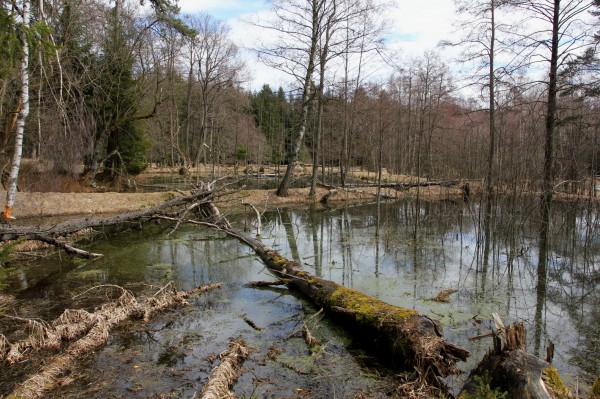
This condition is reasonably well fulfilled in our experiment. It becomes independent on the electron energy E if the dots are separated by a distance shorter than the superconducting coherence length. The latter corresponds to the probability for an electron coming out of one dot so that, instead of escaping into the bulk of the superconducting electrode, it reaches the other dot. Here τ L(R)( E) is the transmission probability of the left (right) quantum dot renormalized by Coulomb interaction, which depends on the energy of an electron and on the side gate potentials applied to the dots V sg,L(R) ( τ L(R)( E) is given by the sum of Lorentzian peaks or Fano resonances associated with discrete energy levels, see Supplementary Note 4, and τ S is the effective transmission probability of the superconducting lead. The ability to tune between the CPS and EC allows for testing of fundamental theoretical concepts relating entanglement and heat transport in the graphene CPS systems. We find that both CPS and EC processes contribute to the non-local thermoelectric current and that their relative contributions can be tuned by the gate potentials. Here we present the experimental observation of the non-local thermoelectric current generated by imposing thermal gradient across a quantum dot–superconductor–quantum dot (QD-S-QD) splitter. The EC and CPS probabilities can in turn be made energy dependent by placing quantum dots between each normal lead and the superconducting region 1, 2. Contrary to intuitive expectations, together these two processes may enable the transfer of heat through the superconductor 3– 5. 4 that the electric transport in the NSN structures depends on the elastic co-tunneling (EC) process on par with the CPS. This connection was further studied and explicitly described for a ballistic NSN structure 4. 29, established a mechanism for the coherent non-local thermoelectric effect in hybrid superconducting systems. The connection between thermoelectric effects and the Cooper pair splitting (CPS) 1, 2, proposed in ref. Thermoelectric effects in the superconducting systems 18– 22, in particular those dealing with non-local thermoelectric currents in superconductor–ferromagnet devices 23– 25 and in bulk non-magnetic hybrid NSN structures 26– 28 have attracted special attention.
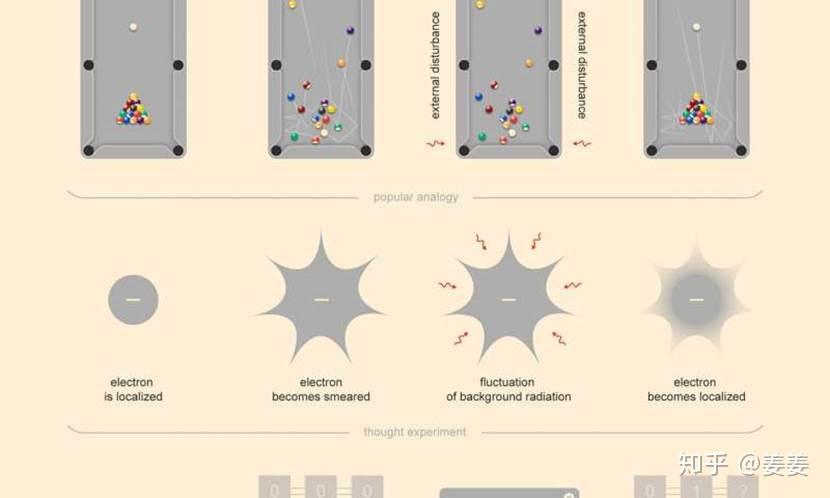
65, 295 (1997).Mesoscopic thermoelectric effects have been investigated in a variety of condensed matter systems that, besides fundamental normal metal–superconductor–normal metal (NSN) systems 1– 5, also include quantum dots 6– 10, atomic point contacts 11– 13, Andreev interferometers 14, 15, superconducting rings 16 and nanowire heat engines 17. Mazenko (World Scientific Press, Singapore, 1986), p. Imry, in Directions in Condensed Matter Physics, edited by G.
